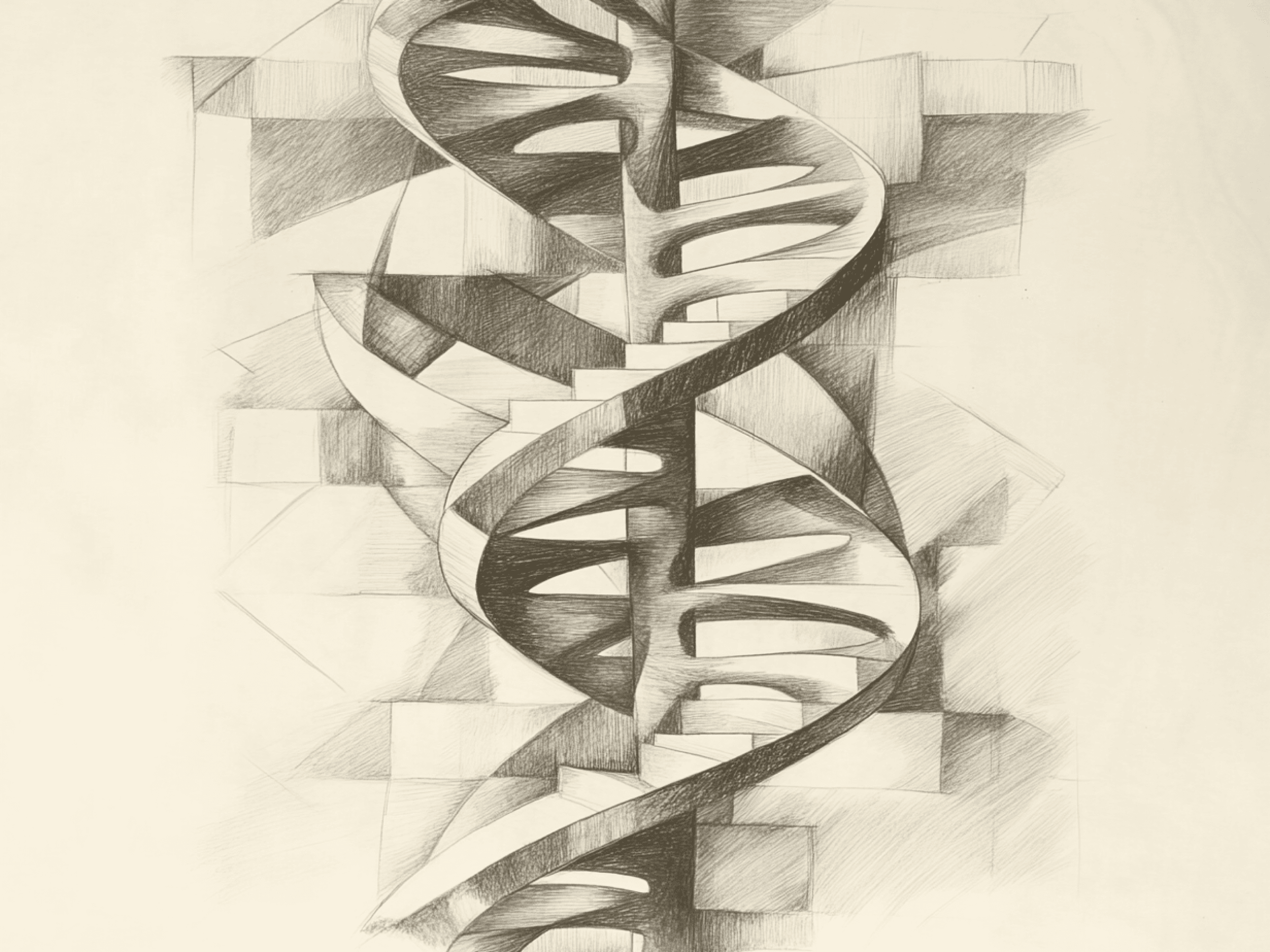
Reproduction is often presented as the ultimate goal of life. This view is a biased projection1 of our condition as a species whose sustainability depends on sexual reproduction. The proposition seems dubious as reproduction is merely a component of a general machine aimed at maintaining the stability of the system—that is, the perpetuation of the species.
Sexual reproduction is just one solution among others. We previously touched on binary fission in bacteria, but life's toolkit also includes mechanisms used by viruses to take over hosts to multiply, budding (for yeasts or hydras), fragmentation, or parthenogenesis. This list is not exhaustive, and these options are not mutually exclusive. Some plants, insects and invertebrates, some reptiles, amphibians, fish, and even a few rare bird species can alternate between sexual reproduction and parthenogenesis. The "best" option seems to depend on environmental factors.
The organism-producing machines are diverse, yet they all involve encoding information, primarily in the form of genes, and interpreting this information to re-produce an organism. We must never lose sight of the fact that these mechanisms, which have evolved over millions of years, exist for one fundamental reason: all organisms die. While we might view the birth of a new organism as the beginning of the life cycle, it is not the cause—death is the cause, and birth is the consequence.
This Darwinian perspective brings us back to Wiener's assertion that "organism is opposed to chaos, to disintegration, to death". Wiener draws an intriguing parallel between the enzymes that enable life through gene expression and Maxwell's demon2, the hypothetical entity that could isolate particles in a closed system, thereby reducing entropy and seemingly contradicting the second law of thermodynamics.
Wiener refutes the possibility of Maxwell's demon by encouraging readers to consider the broader system, which includes the demon itself. He argues that the system's stability is merely a temporary illusion. The demon, through its information processing about the particles it must isolate (a process that cannot be energetically neutral), will eventually accumulate energy and begin to move. Consequently, it will contribute to increasing the entropy of the overall system.
Wiener compares the movement of the demon to the vertigo experienced by Leibniz's monad when its perceptions are altered after receiving an excess of information. The system's temporary stability ultimately depends on the demon's efficiency—its ability to process information while minimizing energy transfer.
While the steps of this demonstration may not be entirely straightforward, the conclusion is universally comprehensible: the stable state of an organism is death. Once life no longer animates an organism, the process of decomposition begins. The train of Nature can be slowed, but it never deviates from its track leading to chaos.
Cybernetics posits that reproduction is not an end in itself, but rather the most effective means of resisting the breakdown of a system—the species—given the limited reliability of its vehicle, the organism. A broad perspective could suggest that no single reproductive mechanism is both necessary and sufficient, as numerous options exist to perpetuate life, and these options are sometimes combined. However, when examining individual species, we often find that a specific reproductive strategy is absolutely necessary.
For instance, in all mammals except humans—an exception we will explore later—the species would cease to exist after a single generation if sexual reproduction were interrupted. This exemplifies a system-machine dependency that has been established through evolution with no possibility of reversal. This dependency serves as a cautionary tale, echoing what we unconsciously experience in our daily lives. However, humans have also managed to consciously exploit this dependency to their advantage.
Today, one of the most ambitious programs to combat malaria relies on the Sterile Insect Technique3 (SIT), which has previously proven successful in the agricultural sector. This method involves releasing large numbers of genetically-modified sterile male insects. Female mosquitoes, unable to distinguish between fertile and sterile males, mate indiscriminately with both. Given the finite number of potential matings in a mosquito's brief lifespan (a single generation lasting only a few days), the presence of sterile males significantly reduces the probability of successful fertilization. This strategy enables the rapid eradication of entire mosquito populations.
At first glance, sexual reproduction doesn’t seem to present so many advantages. How can a process involving two individuals, rather than one, optimize the machinic process? To understand this, let's revisit our earlier definition: the machinic process, regardless of its form, channels energy to resist the increase in entropy. Sexual reproduction raises a significant question in this context: if the objective is the system stability and the process is sexual reproduction, the energy expenditure seems enormous compared to alternatives. Two individuals of opposite sexes must meet (so they must move) at a precise time for fertilization to occur, and the act of mating itself consumes energy. Moreover, we must account for the metabolic cost (indirect) of bearing offspring. Recent research shows that mammals pay the highest reproductive costs (excluding lactation), with approximately 90% of these costs being indirect. Ectotherms (amphibians, reptiles, fish, etc.) expend less energy on reproduction overall, and among them, live-bearing ectotherms incur higher indirect costs compared to egg-layers.
That sexual reproduction established itself as the mechanism for perpetuating the most complex forms of life suggests that its benefits outweigh its higher energy cost in complex organisms. In particular, it must mean that the resulting resistance to entropy is superior to that provided by fission, fragmentation, or parthenogenesis, regardless of the energy loss involved.
To explain this apparent paradox, we must revisit our earlier discussion in the Nature of Disorder on the mutations and insertions that continuously affect the genetic code of all organisms. The optimal mutation rate is not zero due to the energy cost of natural mechanisms to reduce mutations, whether through repair enzymes or by slowing DNA replication. In this context, sexual reproduction proves to be a highly effective solution. It reduces the probability of mutation transmission to the next generation unless the mutation confers decisive advantages.
However, we must also consider another implication of this method, which serves as another caution to those seeking absolute determinism: sexual reproduction is not merely a tool to slow mutations, but it also ensures a minimum level of genetic diversity. Each parent contributes only half of their chromosomes, selected essentially at random.
Inbreeding is generally associated with physical or cognitive developmental issues, and very few species survive under conditions of extreme genetic homogeneity. The most frequently cited example is the vaquita (often called the "sea cow"), one of the world's smallest cetaceans. Its population is limited to only one or two dozen individuals in the Gulf of California, yet it apparently does not suffer from the effects of inbreeding.
While anecdotal, it's worth noting a recent genetic study that provides a fascinating insight into human history. This research suggests that approximately 900,000 years ago, the human species experienced a severe population bottleneck, with the number of breeding individuals reduced to merely 1,300. This critical period lasted about 100,000 years and could have potentially led to the extinction of our species. If we accept the findings of this study, the human exception we are analyzing today might never have come to pass. However, against these odds, humanity not only survived but thrived, ultimately domesticating the natural machines.
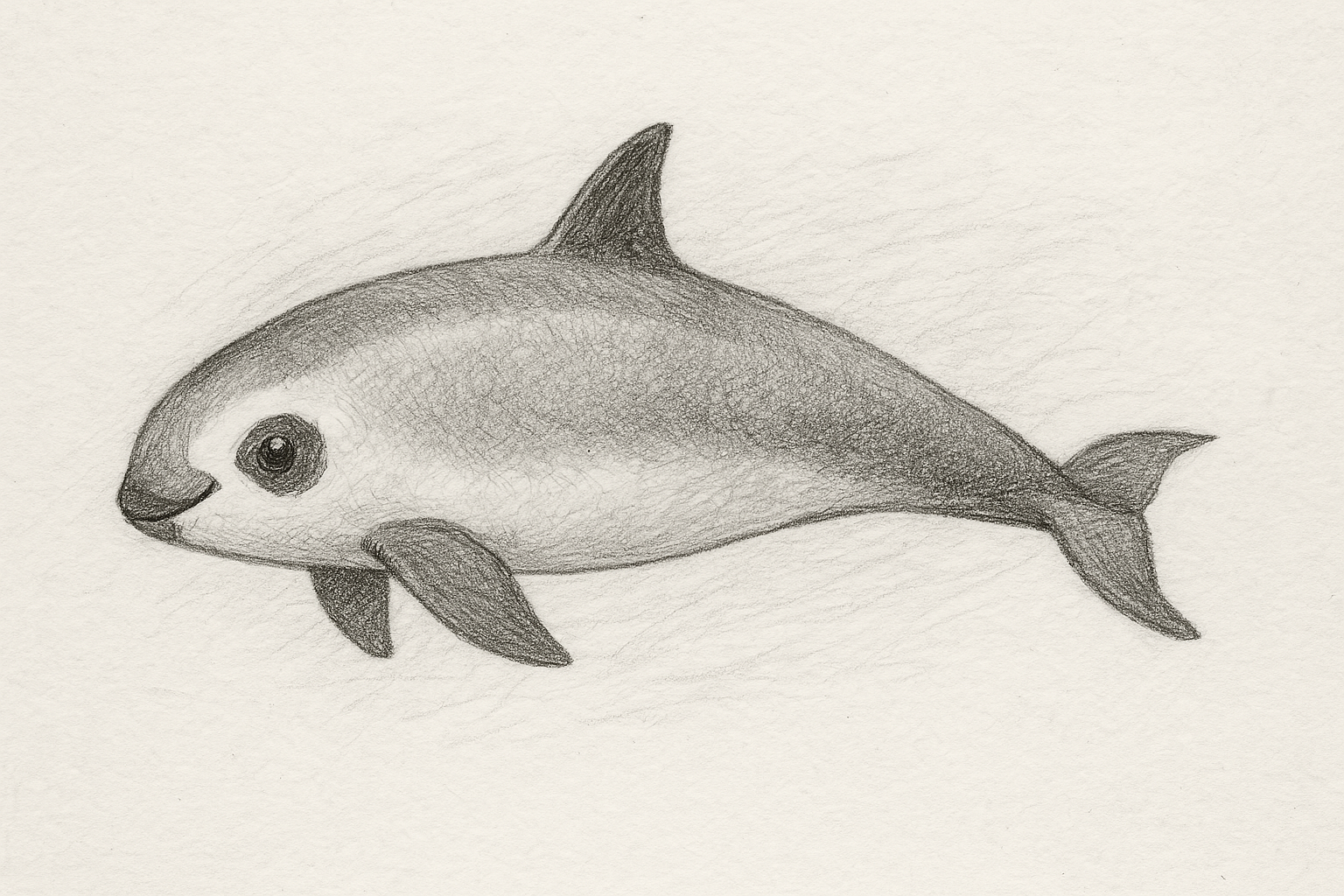
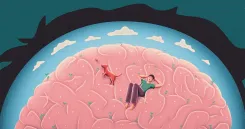
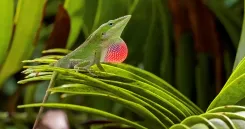
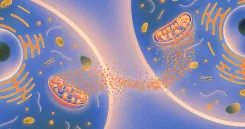